1.1 Introduction
Medical images, such as Computed Axial Tomography (CAT), Magnetic Resonance Imaging (MRI), Ultrasound, and X-Ray, in standard DICOM (Digital Imaging and Communications in Medicine) formats are often stored in Picture Archiving and Communication Systems (PACS) and linked with other clinical information in clinical management systems. Since 70s research efforts have been devoted to processing and analyzing medical images to extract meaningful information such as volume, shape, motion of organs, to detect abnormalities, and to quantify changes in follow-up studies. Automated image segmentation, which aims at automated extraction of object boundary features, plays a fundamental role in understanding image content for searching and mining in medical image archives. A challenging problem is to segment regions with boundary insufficiencies, i.e., missing edges and/or lack of texture contrast between regions of interest (ROIs) and background. To address this problem, several segmentation approaches have been proposed in the literature, with many of them providing rather promising results.
From the literature algorithms designed medical image segmentation are application dependent, imaging modality and type of body part to be studied. For example, requirements of brain segmentation are different from those of the thorax. The artifacts, which affect the brain image, are different partial volume effect is more prominent in brain while in the thorax region it is motion artifact which is more prominent. Thus while selecting a segmentation algorithm one is required to consider all these aspects. The problems common to both CT and MR medical images are:
Partial volume effect
Different artifacts: example motion artifacts, ring artifacts, etc.
Noise due to sensors and related electronic system.
It is well known that there is no a standard algorithm for the segmentation of all medical images. Each imaging system has its own specific limitations. For example, in MR imaging (MRI) one has to take care of bias field noise (intensity inhomogeneities in the RF field). It is obvious that some methods are more general as compared to specialized algorithms, and can be applied to a wider range of data. A brief survey of three generations of medical image segmentation techniques can be found in [].
The motivation of using metaheuristics is to design a new image segmentation techniques, that combine the flexibility of fitness functions with the power of metaheuristics for searching vast search spaces, in order to find the optimal solution. In our work, metaheuristics were improved to solve the continuous and combinatorial optimization problems.
1.2 Image Segmentation as an Optimization Problem
In this section, we show that the segmentation of an image can be reduced to an optimization problem, usually NP-hard []. Hence the need to use a metaheuristic. The segmentation of an image I using a homogeneity feature A is usually defined as a partition
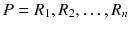
of I , where:

is convex


for all connected regions

.
One can notice that the uniqueness of the segmentation is not guaranteed by these four conditions. Indeed, the segmentation results depend not only on the information contained in the image, but also on the method used to process these information (method used to take a decision looking to the segmentation result). Generally, to reduce the problem of non-uniqueness of the solution, the segmentation problem is regularized by adding an optimization constraint function F characterizing the quality of a good segmentation. Then, a fifth condition is added to the first four:
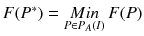
where F is a decreasing function and

is the set of all possible partitions of I .
It is obvious that condition 5 does not entirely solve the problem of uniqueness of the segmentation. There are still cases where multiple segmentations can have the same optimal value. This explains the need to implement algorithms based on metaheuristics.