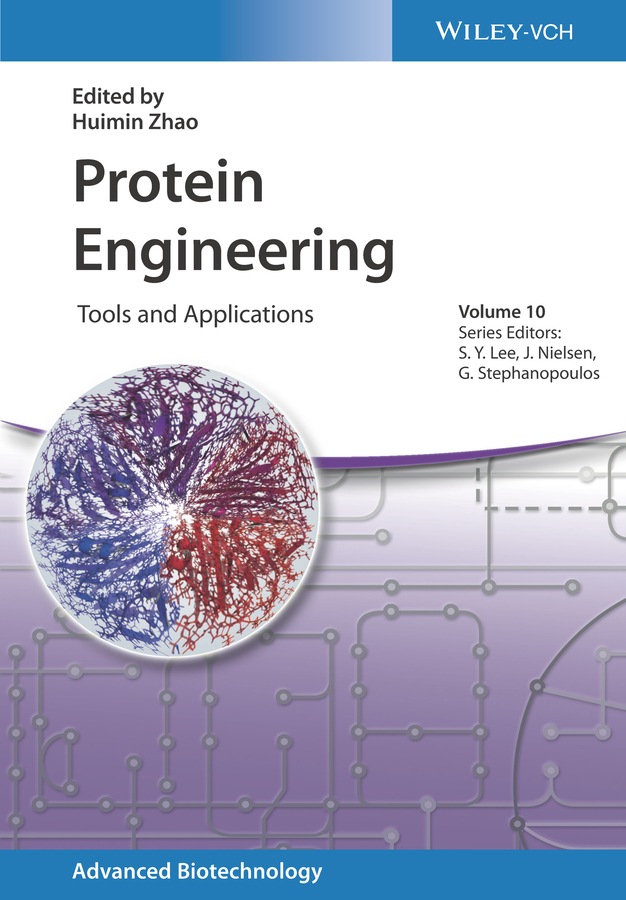
Table of Contents
List of Tables
- Chapter 1
- Chapter 2
- Chapter 3
- Chapter 4
- Chapter 5
- Chapter 7
- Chapter 11
- Chapter 13
List of Illustrations
- Chapter 1
- Chapter 2
- Chapter 3
- Chapter 4
- Chapter 5
- Chapter 6
- Chapter 7
- Chapter 8
- Chapter 9
- Chapter 10
- Chapter 11
- Chapter 12
- Chapter 13
- Chapter 14
- Chapter 15
Guide
Pages
Protein Engineering
Tools and Applications
Edited by
Huimin Zhao

Volume Editor
Professor Huimin Zhao
University of Illinois at Urbana
Chemical & Biomolecular Engineering
600 South Mathews Avenue
215 Roger Adams Laboratory
61801 Urbana IL
USA
Series Editors
Prof. Dr. Sang Yup Lee
KAIST
3731; GuseongDong
291 Daehakro,Yuseonggu
305701 Daejon
South Korea
Prof. Dr. Jens Nielsen
Chalmers University
Department of Biology and Biological Engineering
Kemivgen 10
412 96 Gteborg
Sweden
Prof. Dr. Gregory Stephanopoulos
Massachusetts Institute of Technology
Department of Chemical Engineering
Massachusetts Ave 77
Cambridge, MA 02139
USA
Cover Culture Flasks in microbiological
laboratory / science photo, fotolia
All books published by WILEYVCH are carefully produced. Nevertheless, authors, editors, and publisher do not warrant the information contained in these books, including this book, to be free of errors. Readers are advised to keep in mind that statements, data, illustrations, procedural details or other items may inadvertently be inaccurate.
Library of Congress Card No.:
applied for
British Library CataloguinginPublication Data
A catalogue record for this book is available from the British Library.
Bibliographic information published by the Deutsche Nationalbibliothek
The Deutsche Nationalbibliothek lists this publication in the Deutsche Nationalbibliografie; detailed bibliographic data are available on the Internet at .
2021 WILEYVCH GmbH, Boschstr. 12, 69469 Weinheim, Germany
All rights reserved (including those of translation into other languages). No part of this book may be reproduced in any form by photoprinting, microfilm, or any other means nor transmitted or translated into a machine language without written permission from the publishers. Registered names, trademarks, etc. used in this book, even when not specifically marked as such, are not to be considered unprotected by law.
Print ISBN: 9783527344703
ePDF ISBN: 9783527815098
ePub ISBN: 9783527815111
oBook ISBN: 9783527815128
Cover DesignAdam-Design, Weinheim, Germany
Part I
Directed Evolution
Continuous Evolution of Proteins In Vivo
Alon Wellner1, Arjun Ravikumar1, and Chang C. Liu1,2,3
1University of California, Department of Biomedical Engineering, 3201 Natural Sciences II, Irvine, CA, 92697, USA
2University of California, Department of Chemistry, 1102 Natural Sciences 2, Irvine, CA, 92697, USA
3University of California, Department of Molecular Biology and Biochemistry, 3205 McGaugh Hall, Irvine, CA, 92697, USA
1.1 Introduction
Directed evolution is a powerful approach for engineering new biomolecular and cellular functions [].
To mimic the evolutionary process, classical directed evolution approaches carry out cycles of ex vivo diversification on genes of interest ( GOI s), transformation of the resulting gene libraries into cells, and selection of the desired function ( are capable of generating exceptionally high and precise levels of sequence diversity for any GOI. Second, transforming diversified libraries of the GOI into cells is appropriate, because each GOI variant needs to be translated into a protein in order to express its function, and cells, especially model microbes, are naturally robust hosts for protein expression. Third, carrying out selection inside cells is appropriate, because (i) cells automatically maintain the genotypephenotype connection between the GOI and expressed protein that is necessary for amplification of desired variants, (ii) we often care about a GOIs function within the context of a cell, especially as metabolic engineering and cellbased therapy applications mature, and (iii) the use of cell survival as the output for a desired protein function allows millions or billions of GOI variants to be simultaneously tested it is easy to culture billions of cells under selection conditions in contrast to ex vivo screens that are much lower throughput. Survivalbased selections are not always immediately available, but one can often find a way to reliably link the desired function of a protein to cellular fitness.
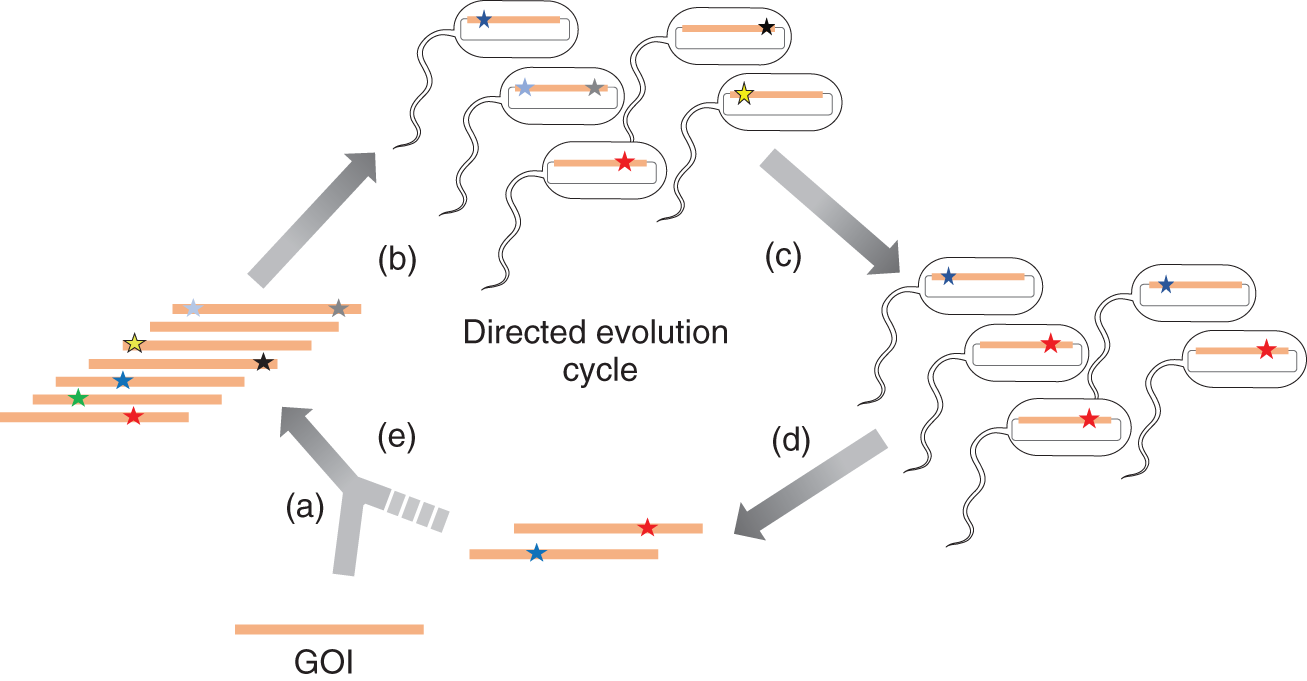
A schematic illustration of a typical directed evolution setup. (a) A GOI is diversified ex vivo, typically by applying an errorprone PCR to generate a GOI library. (b) The library is then cloned into an expression vector and transformed/transfected into cells that are subjected to (c) outgrowth and selection for enhanced protein activity. (d) Plasmid DNA that is enriched for library members with increased properties is extracted and (e) subjected again to diversification and selection. The directed evolution cycle is iterated until the desired outcome is achieved or until diminishing returns (a plateau is reached).
While sensible, the practical requirement that diversification should occur in vitro but expression and selection should occur in vivo in this classical directed evolution pipeline creates significant suboptimalities. First, the number of steps that can be taken along an adaptive path becomes few, since each round of in vitro mutation, transformation, and in vivo selection takes several days or weeks to carry out. Second, limited DNA transformation efficiencies result in strong bottlenecking of diversity that can mitigate the probability of finding the most optimal solutions in sequence space. Third, the number of evolution experiments that can be run simultaneously is minimal, because in vitro mutagenesis, cloning, and transformation are experimentally onerous, demanding extensive researcher intervention . These shortcomings keep two highly promising categories of experiments largely outside the grasp of classical methods: first is the directed evolution of genes towards highly novel functions that likely require long mutational paths to reach (e.g. the optimization of multigene metabolic pathways or the de novo evolution of enzyme activity); and second is the largescale replication of directed evolution experiments, needed in cases when many different functional variants of a gene are desired (e.g. the evolution of multiple synthetic receptors for a collection of ligands) or when statistical power is required in order to understand outcomes in experimental evolution (e.g. probing the scope of adaptive trajectories leading to resistance in a drug target).
An emerging field of in vivo continuous directed evolution seeks to overcome these shortcomings by performing both continuous diversification of the GOI and selection entirely within living cells ]. In this chapter, we discuss various systems that partially or fully achieve
Next page