Other books by this author:
The 23rd Cycle: Learning to Live with a Stormy Star
Patterns in the Void: Why Nothing is Important
The Astronomy Caf: 365 Questions and Answers from Ask the Astronomer
Back to the Astronomy Caf
My Astronomical Life: A first-person journey
Cosmic History II: From the Ice Age to the End of Time
Cosmic History: From the Big Bang to the last Ice Age
Eternity: A Users Guide
Interstellar Travel: An Astronomers Guide
Interplanetary Travel: An Astronomers Guide
Solar Storms: 2000 years of Human Calamity!
Exploring Quantum Space
A Degree in a Book: Cosmology
Space Exploration: A History in 100 objects
Introduction
The investigation of the contents of our universe from the edge of Earths atmosphere to the distant stars and beyond has traditionally been called Astronomy, although prior to the 16th century the term astrology was more commonly applied to this subject. Humans have always gone beyond merely noting the locations of the stars and planets in the sky as they tried to explain what they were seeing but this was usually in terms of the only explanatory framework they knew: mythology or religion.
The character of these explanatory approaches changed dramatically when Johannes Kepler found regularities in the motions of the planets and tried to account for them in terms of various geometric theories. His first two laws were published in 1609 including the first law of elliptical motion. Less than a century later, Sir Isaac Newton succeeded in interpreting the movements of the planets in terms of the action of the force of gravity. This was the first, and very successful, attempt to explain astronomical events and situations in a physics-based theory of how forces interact with matter. In so doing, he created a new sub-discipline in astronomy we now call astrophysics. The most important feature of this new astro-physics was that it was capable of making detailed mathematical predictions of what an astronomer should expect to see if the underlying physical theory and explanation was applicable.
Astrophysics is a subject that develops explanations for astronomical processes and the origins of the major objects in the universe in terms of the actions of forces upon matter, taking full advantage of the detailed mathematics-based theories in physics to show how astronomical systems are formed and evolve through time.
This book will highlight some of the major themes in modern astrophysics. It is a story of not just how things appear in space but why they have taken on these appearances as systems of matter evolving through time.
PART I
The Astrophysicists Toolbox
Chapter 1
Observing the Universe
Since the dawn of the printed word, astronomical instruments have dramatically changed in their accuracy, purpose and appearance. From the simple theodolites and cross-staffs used in the 16th century, to the powerful space telescopes of the 21st century, astronomers have used a variety of tools to help them discover what lies beyond the earth.
THE ELECTROMAGNETIC SPECTRUM
One of the most powerful tools for observing the universe is the electromagnetic (EM) spectrum. The electromagnetic spectrum is a collection of photons sorted according to their increasing wavelength, which can be emitted by objects according to a variety of physical processes. By studying this EM radiation you can diagnose the kinds of physical processes taking place. For example, if a source is a powerful emitter of X-rays, you can tell that it contains very hot gases (called plasma) above temperatures of 100,000 k. If the spectrum follows a curved shape called a black body you can immediately use this fact to take the temperature of the source. If the shape of the spectrum increases sharply to longer wavelengths, this implies there are electrons within the source travelling at nearly the speed of light within strong magnetic fields. Also, if the light appears as discrete, individual lines of emission, you know that the source is a translucent cloud of gas with emission from individual populations of atoms such as calcium, iron, oxygen and so forth.
The types of telescopes used to gather this EM radiation depend on the wavelength of the photons. At optical wavelengths such as those for which our eyes are sensitive near 500 nanometres, simple lenses and mirrors suffice to focus and reflect the EM energy. At much longer wavelengths measured in millimetres and centimetres, you need the technology of radio receivers in which large metallic parabolic dishes are used to focus the radio-wavelength energy.
In addition to detecting faint objects, increasing the aperture of a telescope also greatly improves the resolving power of the system. The basic formula for telescopes is
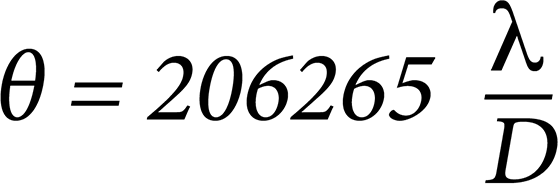
where is the wavelength of light in metres and D is the diameter of the mirror (lens) in metres. The human eye has an aperture of about 5 mm ( in) when fully dark-adapted, so at 500 nm its resolution for = 500 x 10 -9 meters and D=0.005 meters is 30 arcseconds. A 15 cm (6 in) mirror, which is popular for amateur astronomers, can resolve features that are 1 arcsecond in size such as lunar craters 2 km (1 miles) in diameter. However, the turbulence and stability of the atmosphere can limit astronomical seeing to about 1 arcsecond, smearing out details under twinkling starlight. It wasnt until the 1990s when computer and servomotor speeds had greatly improved that this adaptive mirror technique could be widely employed to eliminate atmospheric twinkling. This technique is so effective that modern ground-based telescopes routinely out-perform the space-based Hubble Space Telescope for certain types of observations.
Telescopes as Light Buckets
For millennia, we have learned about the universe by using ordinary human eyesight provided by a 5 mm ( in) lens and an organic photodetector called a retina. But by adding a larger lens or mirror an instrument can be created, which greatly increases the number of photons entering the human eye. The single most important purpose of these instruments, called telescopes, is to collect as many photons of light as possible from distant sources, which is a function often referred to as that of a light bucket. This function is proportional to simply the area of the telescopes primary objective. Large telescope mirrors (and optical apertures generally) increase the amount of light collected from dim objects allowing them to be studied in detail. The aperture of the human eye is only about 5 mm ( in), and allows us to see stars in the sky as faint as the sixth magnitude (+6 m ). By increasing the area of the objective lens or mirror, the brightness limit increases by 5 magnitudes for every 100-fold increase in area. Within the neighbourhood of the sun, most stars are between magnitudes of +6 m and +15 m , while the dimmest stars and galaxies in the visible universe are typically at magnitudes from +20 m to +30 m . To study them we need the largest apertures we can build to gather their faint light, and this is why astronomers are relentlessly building larger telescopes.
Refracting telescopes use a large objective lens at one end of a cylindrical tube, and a set of smaller lenses at the other end of the cylinder called the eyepiece. The Galileo telescope of 1609 had a magnification of about 21 with an objective lens about 37 mm (1 in) in diameter, while the largest refractor at the Yerkes Observatory built in 1895 has an objective lens 102 cm (40 in) diameter. Refracting telescopes of any appreciable size are difficult to make because of the number of optical surfaces that need to be precisely polished to focus light. Also, they are supported around their circumference so the massive objective lens of the Yerkes Refractor, which weighs 250 kg (55 lb), sags at its centre, causing optical changes as the telescope is moved. This limitation is the major reason that the construction of large refractors was abandoned in the 20th century.
Next page