Introduction
The mammalian kidney functions as a key regulator of water balance, acidbase homeostasis, maintenance of electrolytes, and waste excretion. The performance of these activities depends on the development of specific cell types in a precise temporal and spatial pattern, to produce a sufficient number of nephrons. Over the past several decades, considerable advances have been made in understanding the molecular basis for this developmental program. Defects in this program result in congenital anomalies of the kidney and urinary tract, which are the leading causes of chronic kidney disease and renal failure in children. These developmental disorders range from renal malformations, such as renal aplasia (absence of the kidney), dysplasia (failure of normal renal differentiation), and hypoplasia (smaller kidneys), to urinary tract abnormalities such as vesicoureteral reflux and duplicated collecting systems. This chapter describes the embryology of the kidney and urinary tract, as a means to understand the developmental origins of these disorders.
Human kidney development starts in the fifth week of gestation, and new nephrons are formed until approximately 3234 weeks gestation [].
Critical determinants of nephron endowment are structural development and three-dimensional nephron patterning. The formation of kidneys in utero involves the coordinated regulation of critical developmental processes: differentiation, morphogenesis, and regulation of cell number. Differentiation is the process by which precursor cells or tissues mature into more specialized cells. During kidney development, renal mesenchymal cells have the potential to differentiate into nephron epithelia or stromal cells and interstitial fibroblasts. Morphogenesis describes the process whereby cells and tissues acquire three-dimensional patterns. This is particularly important in the kidney, as the three-dimensional relationship between the nephrons, the vasculature, and the collecting system is critical for normal kidney structural function. Finally, the regulation of cell number at different stages of development is crucial. Such regulation maintains a balance between cellular proliferation and programmed cell death or apoptosis. All of these processes are integrated tightly and regulated both spatially and temporally in normal renal development.
The molecular control of these developmental processes has been the subject of several recent comprehensive reviews [].
Studying the Kidney and Urinary Tract Development
The methods for studying the molecular and genetic control of kidney development have continued to evolve over the past several decades. Visualization of tissue morphology and expression of individual genes and proteins in the developing kidney have traditionally been performed on tissue sections by general staining (e.g., hematoxylin and eosin), detecting messenger RNA (mRNA) via in situ hybridization or protein via immunohistochemistry (Fig. ].
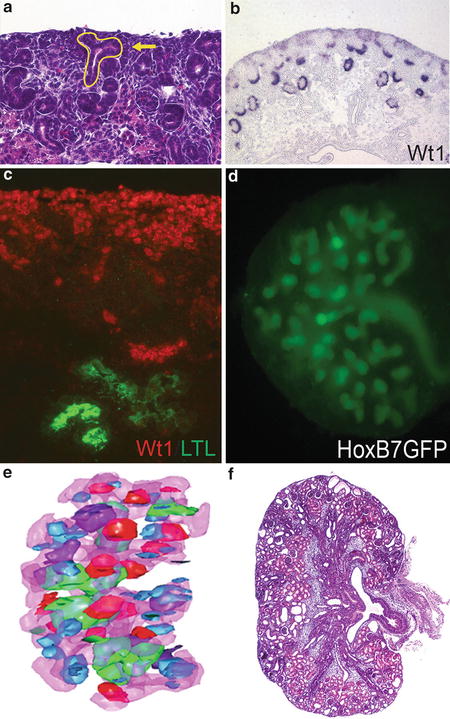
Fig. 1
Experimental methods utilized to study kidney development . ( a ) Hematoxylin and eosin ( H&E )-stained tissue section of a control postnatal day 0 mouse kidney. The ureteric bud is outlined in yellow , and the arrow points to the cap metanephric mesenchyme. ( b ) In situ hybridization in a control mouse embryonic day 16.5 tissue section for the transcription factor, Wt1 , which stains the metanephric mesenchyme and developing glomeruli. ( c ) Immunofluorescent staining in a control embryonic day 14.5 mouse tissue section for the transcription factor, Wt1 ( red ), and lotus tetragonolobus lectin ( LTL , green ), which stains the proximal tubule. ( d ) Embryonic culture of a transgenic embryonic day 11.5 HoxB7GFP mouse kidney, demonstrating branching ureteric structures ( green ) after 5 days of growth. ( e ) 3D reconstruction of an embryonic day 13.5 mouse kidney with the ureteric epithelium depicted in pink and developing nephron types including renal vesicles ( blue ), comma-shaped bodies ( red ), S-shaped bodies ( purple ), and glomeruli ( green ) (Reproduced with kind permission from Springer Science+Business Media: Sims-Lucas S. Kidney Development: Methods and Protocols, Methods in Molecular Biology, vol. 886, 2012, pp 81, Figure ) ( f ) H&E-stained tissue section of a postnatal day 0 mouse kidney lacking microRNAs in the ureteric lineage using a conditional knockout approach ( HoxB7Cre; Dicer flx/flx )
A classical technique to analyze kidney development is to culture rodent embryonic kidneys in vitro as explants. Studies using these methods were the first to show that reciprocal interactions between the metanephric mesenchyme and the ureteric bud are critical to induce the formation of new nephrons and ureteric branching (Fig. ]. While these experiments have been illuminating, the growth of embryonic kidney explants differs from kidney development in vivo in several key ways: lack of blood flow, growth limitations from diffusion of the culture media across the airmedia interface, and distortions in the three-dimensional kidney architecture as explants flatten in culture.
Recently, several methods to generate more physiological and quantifiable three-dimensional reconstructions of developing kidneys and urinary tracts have been developed. One method utilizes exhaustive serial sectioning through developing kidneys, histological staining, projection of each serial image onto a monitor to identify each tissue lineage, and rendering of the serial images into a three-dimensional image (Fig. ].
Physical, chemical, and genetic strategies can be used to manipulate developing kidneys in vivo. For example, ureteric obstruction in utero in sheep and monkeys results in hydronephrotic kidneys with renal dysplasia []. Transgenic approaches have also been used to drive gene expression in specific spatiotemporal patterns, usually in the mouse. In these experiments, a transgenic construct consisting of a tissue-specific promoter and the gene of interest is randomly inserted into the genome, leading to expression of that gene in a tissue-specific pattern. The limitations of this approach include: (1) that the random insertion can result in unintended changes in gene expression (due to other nearby promoters/enhancers near the site of integration), (2) that the insertion of the transgene into the genome may lead to loss of function of an endogenous gene, and that (3) epigenetic factors may silence the construct. The increased utilization of bacterial artificial chromosome (BAC) constructs, which contain more of endogenous promoter elements than are found in traditional plasmid constructs, has led to more faithful and reliable transgene expression.
As opposed to transgenic approaches, homologous recombination is the method whereby a gene is knocked-out of or knocked-into the mouse genome. Using these methods, a gene of interest is deleted so that it becomes nonfunctional, or a gene (such as a green fluorescent reporter) is added to the genome at a specific locus. A limitation to traditional knockout techniques is that global loss of function of the gene may result in extrarenal effects (such as early embryonic lethality), which can impact or severely limit the study of the genes function in the kidney. Given these limitations, it has become more common to perform conditional gene targeting (e.g., with the CreloxP system) (Fig. ) and/or inducible gene targeting (e.g., with tamoxifen), allowing for kidney- and/or urinary tract-specific gene deletion (using a kidney zebrafish specific Cre-) and/or at a particular time (driving induction of gene targeting with a drug).