Published by GENERAL PRESS 4805/24, Fourth Floor, Krishna House Ansari Road, Daryaganj, New Delhi 110002 Ph. : 011 23282971, 45795759 e-mail : generalpressindia@gmail.com www.generalpress.in General Press All rights reserved. No part of this publication may be reproduced, stored in a retrieval system, or transmitted, in any form or by any meanselectronic, mechanical, photocopying, recording or otherwisewithout the prior written permission of the publishers. First Edition : 2018 ISBN : 9789388118491 3 0 1 0 2 0 1 8 |
Contents
To
QUIGG NEWTON
who reads all my books
Preface
Gravity rules the Universe. It holds together the one hundred billion stars of our Milky Way; it makes the Earth revolve around the Sun and the Moon around the Earth; it makes ripened apples and disabled airplanes fall to the ground. There are three great names in the history of mans understanding of gravity: Galileo Galilei, who was the first to study in detail the process of free and restricted fall; Isaac Newton, who first had the idea of gravity as a universal force; and Albert Einstein, who said that gravity is nothing but the curvature of the four-dimensional space-time continuum.
In this book we shall go through all three stages of the development, devoting one chapter to Galileos pioneering work, six chapters to Newtons ideas and their subsequent development, one chapter to Einstein, and one chapter to post-Einsteinian speculations concerning the relation between gravity and other physical phenomena. The emphasis on the classics in this outline grows from the fact that the theory of universal gravity is a classical theory. It is very probable that there is a hidden relation between gravity on the one hand and the electromagnetic field and material particles on the other, but nobody is prepared today to say what kind of relation it is. And there is no way of fore-telling how soon any further important progress will be made in this direction.
Considering the classical part of the theory of gravitation, the author had to make an important decision about the use of mathematics. When Newton first conceived the idea of Universal Gravity, mathematics was not yet developed to a degree that could permit him to follow all the astronomical consequences of his ideas. Thus Newton had to develop his own mathematical system, now known as the differential and integral calculus, largely in order to answer the problems raised by his theory of universal gravitation. Therefore it seems reasonable, and not only from the historical point of view, to include in this book a discussion of the elementary principles of calculus, a decision which accounts for a rather large number of mathematical formulas in the third chapter. The reader who has the grit to concentrate on that chapter will certainly profit by it as a basis for his further study of physics. On the other hand, those who are frightened by mathematical formulas can skip that chapter without much damage to a general understanding of the subject. But if you want to learn physics, please do try to understand Chapter 3!
George Gamow
University of Colorado
January 13, 1961
1. How Things Fall

The notion of up and down dates back to time immemorial, and the statement that everything that goes up must come down could have been coined by a Neanderthal man. In olden times, when it was believed that the world was flat, up was the direction to Heaven, the abode of the gods, while down was the direction to the Underworld. Everything which was not divine had a natural tendency to fall down, and a fallen angel from Heaven above would inevitably finish in Hell below. And, although great astronomers of ancient Greece, like Eratosthenes and Aristarchus, presented the most persuasive arguments that the Earth was round, the notion of absolute up-and-down directions in space persisted through the Middle Ages and was used to ridicule the idea that the Earth could be spherical. Indeed, it was argued that if the Earth were round, then the antipodes, the people living on the opposite side of the globe, would fall off the Earth into empty space below, and, far worse, all ocean water would pour off the Earth in the same direction.
When the sphericity of the Earth was finally established in the eyes of everyone by Magellans round-the-world trip, the notion of up-and-down as an absolute direction in space had to be modified. The terrestrial globe was considered to be resting at the center of the Universe while all the celestial bodies, being attached to crystal spheres, circled around it. This concept of the Universe, or cosmology, stemmed from the Greek astronomer Ptolemy and the philosopher Aristotle. The natural motion of all material objects was toward the center of the Earth, and only Fire, which had something divine in it, defied the rule, shooting upward from burning logs. For centuries Aristotelian philosophy and scholasticism dominated human thought. Scientific questions were answered by dialectic arguments (i.e., by just talking), and no attempt was made to check, by direct experiment, the correctness of the statements made. For example, it was believed that heavy bodies fall faster than light ones, but we have no record from those days of an attempt to study the motion of falling bodies. The philosophers excuse was that free fall was too fast to be followed by the human eye.
The first truly scientific approach to the question of how things fall was made by the famous Italian scientist Galileo Galilei (15641642) at the time when science and art began to stir from their dark sleep of the Middle Ages. According to the story, which is colorful but probably not true, it all started one day when young Galileo was attending a Mass in the Cathedral of Pisa, and absent-mindedly watched a candelabrum swinging to and fro after an attendant had pulled it to the side to light the candles (Fig. 1). Galileo noticed that although the successive swings became smaller and smaller as the candelabrum came to rest, the time of each swing (oscillation period) remained the same. Returning home, he decided to check this casual observation by using a stone suspended on a string and measuring the swing period by counting his pulse. Yes, he was right; the period remained almost the same while the swings became shorter and shorter. Being of an inquisitive turn of mind, Galileo started a series of
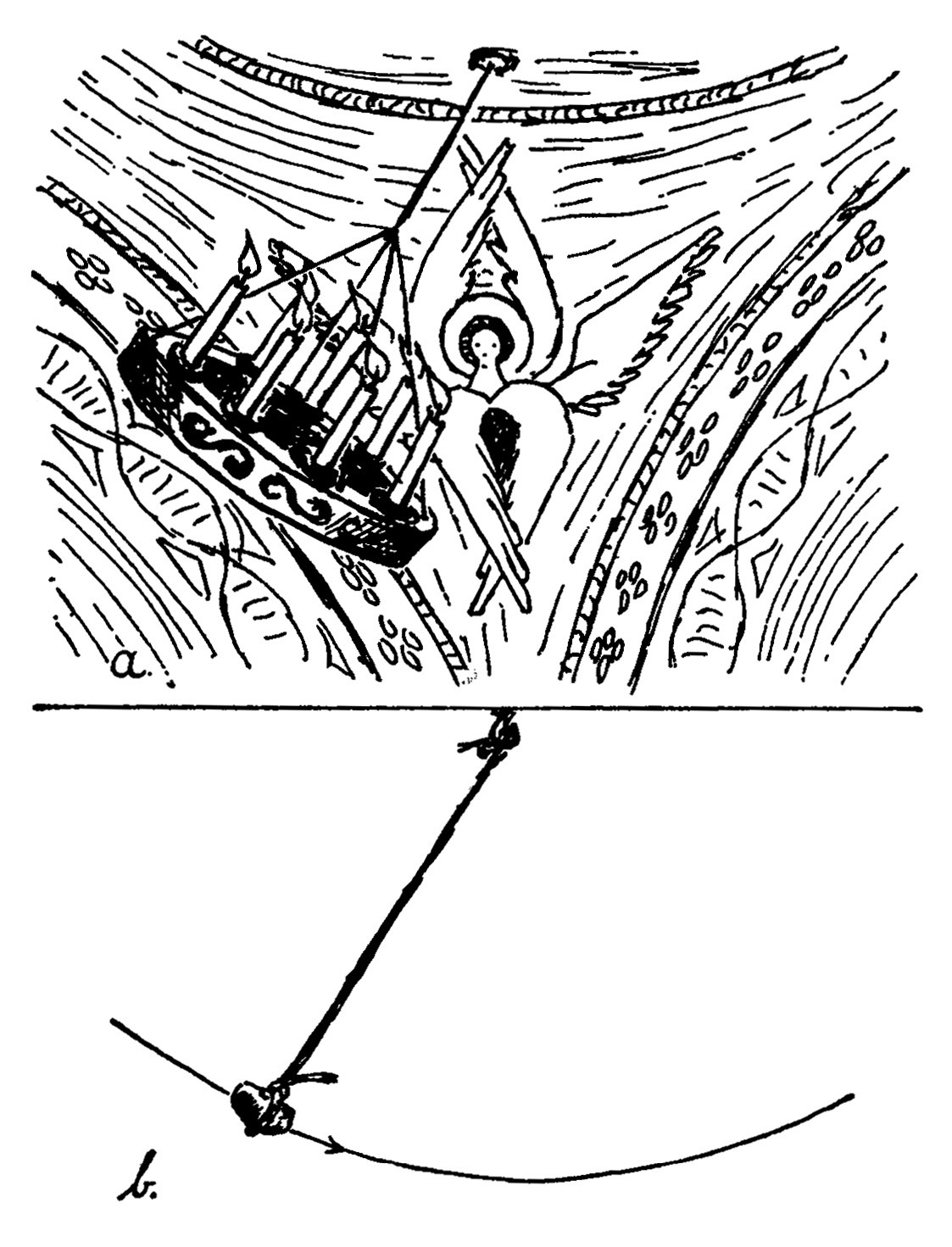
Fig. 1. A candelabrum (a) and a stone on a rope (b) swing with the same period if the suspensions are equally long.
experiments, using stones of different weights and strings of different lengths. These studies led him to an astonishing discovery. Although the swing period depended on the strings length (being longer for longer strings), it was quite independent of the weight of the suspended stone. This observation was definitely contradictory to the accepted dogma that heavy bodies fall faster than light ones. Indeed, the motion of a pendulum is nothing but the free fall of a weight deflected from a vertical direction by a restriction imposed by a string, which makes the weight move along an arc of a circle with the center in the suspension point (Fig. 1).