Part I
Introduction
International Atomic Energy Agency 2017
Joanna Jankowicz-Cieslak , Thomas H. Tai , Jochen Kumlehn and Bradley J. Till (eds.) Biotechnologies for Plant Mutation Breeding 10.1007/978-3-319-45021-6_1
1. Mutagenesis for Crop Breeding and Functional Genomics
Joanna Jankowicz-Cieslak 1, Chikelu Mba 2 and Bradley J. Till 1
(1)
Plant Breeding and Genetics Laboratory, Joint FAO/IAEA Division of Nuclear Techniques in Food and Agriculture, IAEA Laboratories Seibersdorf, International Atomic Energy Agency, Vienna International Centre, 100, 1400 Vienna, Austria
(2)
Seeds and Plant Genetic Resources Team, Plant Production and Protection Division, Food and Agriculture Organization of the United Nations, Rome, Italy
Abstract
Genetic variation is a source of phenotypic diversity and is a major driver of evolutionary diversification. Heritable variation was observed and used thousands of years ago in the domestication of plants and animals. The mechanisms that govern the inheritance of traits were later described by Mendel. In the early decades of the twentieth century, scientists showed that the relatively slow rate of natural mutation could be increased by several orders of magnitude by treating Drosophila and cereals with X-rays. What is striking about these achievements is that they came in advance of experimental evidence that DNA is the heritable material. This highlights one major advantage of induced mutations for crop breeding: prior knowledge of genes or gene function is not required to successfully create plants with improved traits and to release new varieties. Indeed, mutation induction has been an important tool for crop breeding since the release of the first mutant variety of tobacco in the 1930s. In addition to plant mutation breeding, induced mutations have been used extensively for functional genomics in model organisms and crops. Novel reverse-genetic strategies, such as Targeting Induced Local Lesions IN Genomes (TILLING), are being used for the production of stable genetic stocks of mutant plant populations such as Arabidopsis , barley, soybean, tomato and wheat. These can be kept for many years and screened repeatedly for different traits. Robust and efficient methods are required for the seamless integration of induced mutations in breeding and functional genomics studies. This chapter provides an overview of the principles and methodologies that underpin the set of protocols and guidelines for the use of induced mutations to improve crops.
Keywords
Mutation breeding Reverse-genetics Forward-genetics Phenotyping Genotyping Technology packages
1.1 Inducing Genetic Variation
The genetic improvement of crops is a crucial component of the efforts to address pressures on global food security and nutrition (Ronald ).
Fig. 1.1
Crop improvement strategies based on the generation and harnessing of genetic variation. There are many methods to introduce novel genetic variation into a specific line. The most common is through outcrossing, whereby introgression and recombination generate new combinations of alleles. This may include wide intraspecific and interspecific crosses. Passaging of cells through tissue culture has also been used to generate what is known as somaclonal variation. Alter by design refers to any method whereby genetic variation is induced through thoughtful modifications. These include methods such as transgenics or genome editing (see Chap. )
1.1.1 Practical Considerations in Induced Crop Mutagenesis
Mutation breeding is a three-step process consisting of (a) inducing mutations, (b) screening for putative mutant candidates and (c) mutant testing and official release (Fig. ).
Fig. 1.2
A three-step mutation breeding scheme for direct release of improved crops. Each part is drawn proportional to the estimated time needed for development of a seed-propagated cereal (710 years). The first step is mutation induction which may take up to a year. The most time consuming and complicated step is mutant selection. Several years are typically needed to identify useful traits that are stable through propagation cycles. The third step, mutant varietal release, follows standardised procedures of the country where the material is grown. This often requires multilocational trials with farmer involvement. While the timing of this may vary, it is usually a shorter duration than the selection and testing phase. The procedure becomes longer and more complicated if the selected mutants are used as pre-breeding material in hybridisations (see Chap. )
The choice of which type of mutagen to use for mutation breeding is often based on past successes reported for the species and other considerations such as the availability of mutagens, costs and infrastructure (Bado et al. of this book describe chemical and/or physical mutagenesis protocols for obligate vegetatively propagated banana ( Musa acuminata ), facultative vegetatively propagated Jatropha ( Jatropha curcas ) and seed-propagated barley ( Hordeum vulgare ).
A major bottleneck in plant mutation breeding is the imperative of generating and evaluating large mutant populations in order to increase the chance of identifying a desirable variant. Efforts are devoted to the dissociation of chimeras, also known as mosaics or sectoral differences, whereby cells of different genotypes exist side by side in the tissues of the same mutant plant. This is straightforward in sexually produced crops owing to the fact that single cells in the form of gametes are the basis for the next generation, thus resolving any chimeras. For vegetatively propagated crops, several cycles of regeneration may be required to produce solid homohistonts or genotypically homogeneous material (van Harten . These approaches have been less often used than those involving multicellular organs and tissues, and so there is less information available on the possibility of chimerism at the DNA sequence. It is interesting to speculate on the fate of induced DNA modifications in single cells. For example, EMS mutagenesis results in alkylation, whereby the original base is not physically altered, but the mutation is only fixed due to an error in replication of the affected base. Here, two daughter cells could be produced with distinct genotypes.
1.1.2 Developing Crop Varieties Using Induced Mutations
Once a mutant population has been developed, the next steps of the mutation breeding process mirror traditional breeding procedures (Fig. ).
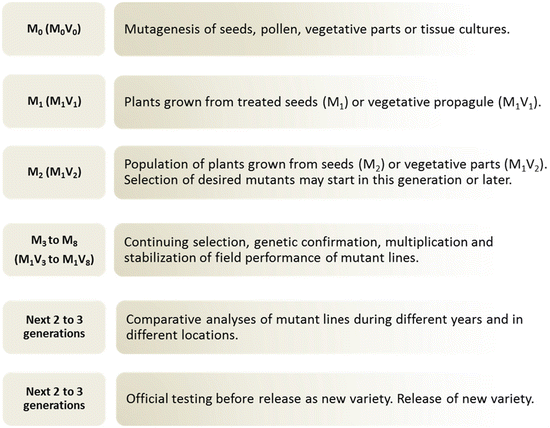
Fig. 1.3
Traditional mutation breeding scheme. Each row describes the steps for a specific generation. The generation nomenclature starts with M0 for seed or pollen mutagenesis and M0V0 for vegetative organs, where M stands for the meiotic and V for the vegetative generation. All materials are labelled with a 0 prior to mutagenesis and with a 1 after mutagenesis is performed. The first generation is not suitable for evaluation when multicellular material is mutagenised because resulting plants will be genotypically heterogeneous (chimeric). The first non-chimeric (homohistont) generation in a seed-mutagenised and seed-propagated material is the M2. It may take several cycles to make a vegetatively propagated material genotypically homogeneous and to stabilise the inheritance of mutant alleles. Screening and selections can begin as early as the first non-chimeric generation. Subsequent generations typically involve selection and evaluation of mutant phenotypes to ensure that the traits are reproducible. Once this is complete, the materials can enter trials for varietal release. Alternatively, materials can be used as parents in breeding programmes. Officially released mutant crop varieties which are reported to the Joint FAO/IAEA Programme are recorded in the searchable Mutant Variety Database (MVD )
Next page