Contents
Guide
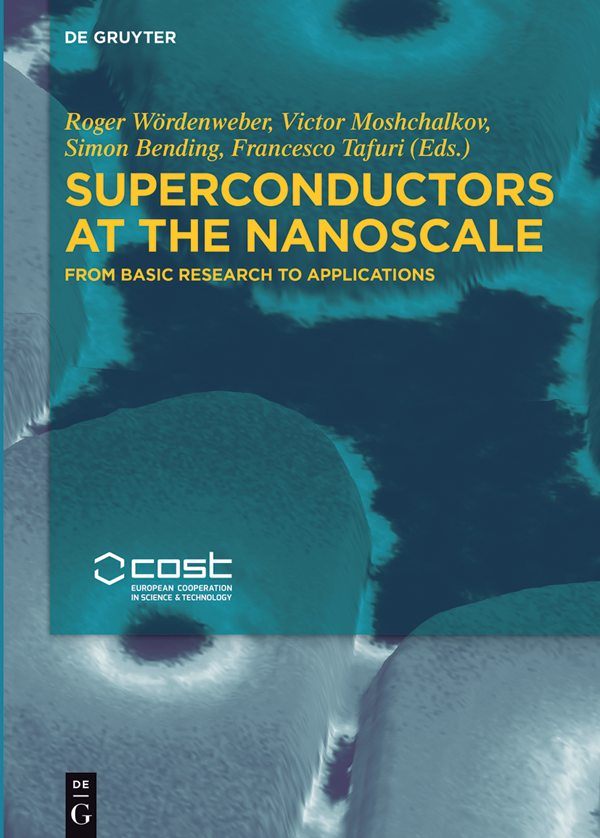
Roger Wrdenweber, Victor Moshchalkov, Simon Bending and Francesco Tafuri
Superconductors at the Nanoscale
Also of Interest
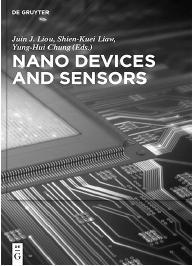
Nano Devices and Sensors
Juin J. Liou, Shien-Kuei Liaw, Yung-Hui Chung, 2016
ISBN 978-1-5015-1050-2, e-ISBN 978-1-5015-0153-1
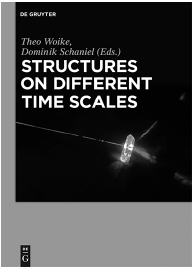
Structures on Time Scales
Theo Woike, Dominik Schaniel, 2017
ISBN 978-3-11-044209-0, e-ISBN 978-3-11-043392-0
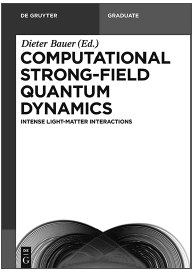
Computational Strong-Field Quantum Dynamics. Intense Light-Matter
Interactions
Dieter Bauer, 2017
ISBN 978-3-11-041725-8, e-ISBN 978-3-11-041726-5
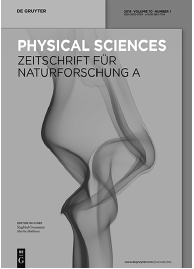
Zeitschrift fr Naturforschung A. A Journal of Physical Sciences
Martin Holthaus (Editor-in-Chief)
ISSN 0932-0784, e-ISSN 1865-7109
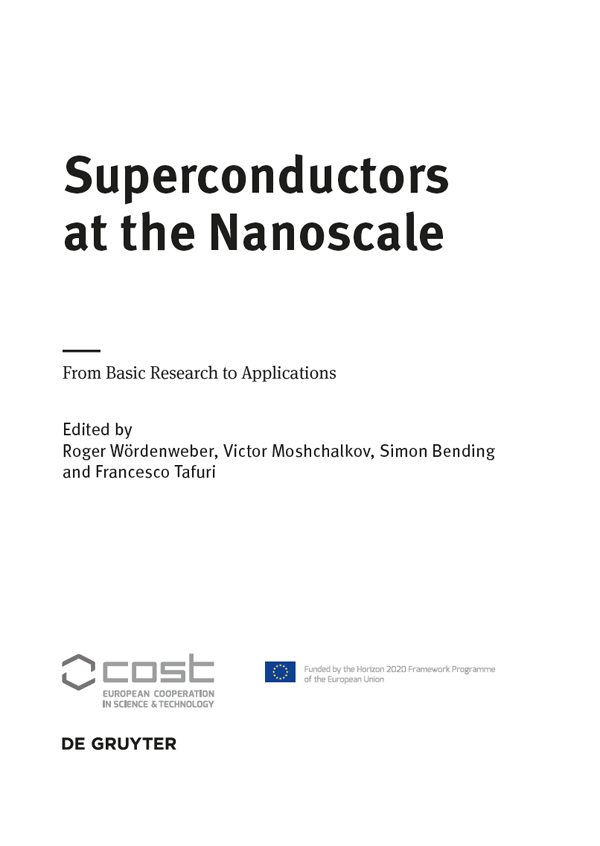
Editors
Prof. Dr. Roger Wrdenweber
Forschungszentrum Jlich
Peter Grnberg Institut (PGI-8)
52425 Jlich
Germany
Prof. Victor Moshchalkov
KU Leuven
Institute for Nanoscale Physics
and Chemistry
Celestijnenlaan 200D
3001 Heverlee
Belgium
Prof. Simon Bending
University of Bath
School of Physics
Claverton Down
Bath Ba2 7AY
United Kingdom
Prof. Francesco Tafuri
Seconda Universit di Napoli
Via Roma 29
81031 Aversa
Italy
Cover Image: Artistic 3D view (realized by Dr. T. Cren INSP, Sorbonne Universits, CNRS, Paris, France) of quantum vortices in superconducting nano-islands of Pb subject to a magnetic field. Individual Abrikosov-Pearl vortices appear as regular dark spots inside the islands and the Josephson ones in between (see D. Roditchev, et al. Nature Phys. 11, 332 (2015) and in this book: STM studies of vortex cores in strongly confined nanoscale superconductors ).
ISBN 978-3-11-045620-2
e-ISBN (PDF) 978-3-11-045680-6
e-ISBN (EPUB) 978-3-11-045624-0
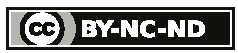
This work is licensed under the Creative Commons Attribution-NonCommercial-NoDerivs 3.0 License. For details go to http://creativecommons.org/licenses/by-nc-nd/3.0/.
Library of Congress Cataloging-in-Publication Data
A CIP catalog record for this book has been applied for at the Library of Congress.
Bibliographic information published by the Deutsche Nationalbibliothek
The Deutsche Nationalbibliothek lists this publication in the Deutsche Nationalbibliografie; detailed bibliographic data are available on the Internet at http://dnb.dnb.de.
2017 published by Walter de Gruyter GmbH, Berlin/Boston
The book is published with open access at www.degruyter.com.
Cover image: Drs. Ch. Brun, T. Cren, and D. Roditchev INSP, Sorbonne Universits, CNRS, Paris, France
www.degruyter.com
Foreword
The enigmatic problem of perpetuum mobile has attracted a lot of attention over the years, starting already in the Middle Ages. Indeed, perpetual motion implies a lack of energy dissipation which is a very unusual situation in science. Two key cases of nondissipating motion on a macroscopic scale are well known:
the flow of electrical current in superconductors and
the propagation of light (and other electromagnetic waves as well) in vacuum .
If a current is induced in a superconducting ring that is meters or kilometers in size, it circulates there forever. When we enjoy the romantic glimmer of a distant star in the night, the light from it has arrived after traveling for billions of years, a nice experimental proof of dissipation-free propagation. An important difference here is that the first system deals with current in condensed matter , the second one with the propagation of electromagnetic fields in vacuum . In the first case, the energy dissipation is forbidden by the existence of the coherent quantum state of the condensate of the charged Cooper pairs carrying the current, while in the second case there is not too much to interact with for the light propagating in vacuum, as prescribed by the classical Maxwells equations.
Whereas propagating light interacts with matter or gravitational waves and represents the basis for optical devices and experiments, the frictionless flow of supercurrent interferes with nanosize objects in the superconductor such as tunnel barriers, surfaces, interfaces, or the so-called fluxons or vortices , quantized magnetic flux of extremely small magnitude = h /2 e 2.06 10 Wb, that are induced by an applied current, a magnetic field, or thermal fluctuations. On the one hand, an appropriate nanotechnology is required to master fluxon behavior for instance through designing appropriate pinning potentials to localize the fluxons (vortices) and retain the frictionless supercurrent that is necessary for a number of superconducting applications. This forms one of the main objectives of fluxonics . On the other hand, it offers a wide range of options for improved or even novel fluxonic concepts, especially since the necessary tools for nanoengineering superconducting materials are readily available nowadays.
Generally, the superconducting condensate is described by the order parameter that obeys the GinzburgLandau (GL) equations ( Nobel Prize in Physics, 2003 ). The boundary conditions for these, strongly influencing the solutions, are imposed at the physical sample boundaries, thus implying that the properties of confined fluxons can be tailored by applying specific surface configurations. This creates a unique opportunity for the quantum design of the physical properties of the confined condensates and fluxons through the application of specially defined nanomodulated boundary conditions, which can be additionally tuned using, for instance, magnetic templates, electrical fields, or even optical signals. The imposed nanomodulation can therefore lead to the practical implementation of the confined fluxon patterns possessing the specific properties needed for applications in fluxonics ranging from passive and active elements to qubits for quantum computing.
It is the intention of this book to highlight and discuss the state-of-the-art and recent progress in this field, as well as to highlight current problems with Superconductors at the Nanoscale. This includes:
the visualization and understanding of fluxons (vortices) and their interaction on the nanoscale, in nanostructured superconductors, as well as in novel types of superconductors;
progress in controlling static fluxon configurations as well as the dynamic properties (up to THz frequencies) of fluxons in nanoscale superconductors;
the behavior of different types of fluxons (Abrikosov vortices, kinematic vortices, and Josephson vortices) inmesoscopic, nanostructured, and/or layered superconductors;