Lee - Molecular Mechanisms of Learning and Memory
Here you can read online Lee - Molecular Mechanisms of Learning and Memory full text of the book (entire story) in english for free. Download pdf and epub, get meaning, cover and reviews about this ebook. year: 2010, publisher: Web Books Publishing, genre: Children. Description of the work, (preface) as well as reviews are available. Best literature library LitArk.com created for fans of good reading and offers a wide selection of genres:
Romance novel
Science fiction
Adventure
Detective
Science
History
Home and family
Prose
Art
Politics
Computer
Non-fiction
Religion
Business
Children
Humor
Choose a favorite category and find really read worthwhile books. Enjoy immersion in the world of imagination, feel the emotions of the characters or learn something new for yourself, make an fascinating discovery.
Molecular Mechanisms of Learning and Memory: summary, description and annotation
We offer to read an annotation, description, summary or preface (depends on what the author of the book "Molecular Mechanisms of Learning and Memory" wrote himself). If you haven't found the necessary information about the book — write in the comments, we will try to find it.
Lee: author's other books
Who wrote Molecular Mechanisms of Learning and Memory? Find out the surname, the name of the author of the book and a list of all author's works by series.
Molecular Mechanisms of Learning and Memory — read online for free the complete book (whole text) full work
Below is the text of the book, divided by pages. System saving the place of the last page read, allows you to conveniently read the book "Molecular Mechanisms of Learning and Memory" online for free, without having to search again every time where you left off. Put a bookmark, and you can go to the page where you finished reading at any time.
Font size:
Interval:
Bookmark:
Albert Einstein said, "The most incomprehensible fact about nature is that itis comprehensible." Indeed, physical phenomena are comprehensible.Weather changes, chemical reactions and molecular structures appear quite complex, but their underlying principles are amazingly simple. All physical phenomena are governed by four fundamentalforces - gravity, electromagnetism, strong and weak nuclear forces. Recently, these forcescan further be unified by a single theory -- the string theory.
What about the brain? Is its ability of comprehension comprehensible? In the seventeenth century, Isaac Newton had discovered the gravitational law and Robert Boyle had formulated the equation for gas properties, but human beings were still quite ignorant about physiological systems. William Harvey, a pioneer in blood circulation, told Robert Boyle that it was the valves in the veins which guided him to the next phase of his thinking.
We now know that thoughts are not controlled by blood vessels and sorrow has nothing to do with a "broken heart". They come from our brain -- the onlything which can try to understanditself. With a size smaller than a microwave oven, our brain contains more than 10 billions of neurons. Each neuron is connected to many (up to thousands) other neurons. The junction between neurons is calleda synapse. Brain functions are the results of signal transmission through trillions of synapses.
Since neurons are made up of the same atoms as found in the physical world, it might be possible to explain brain functions in terms of physical laws. In thepast few decades, significant progress has been made toward this goal. The formation of short-term memory through learning has been well understood.However, conversion from short-term to long-term memory is still a mystery. In Chapters 9 and 10, we shall present a possible mechanism which involves microtubules.
Nervous systems, like other communication systems, use a sequence of impulses to carry message. The nature of nerve impulses, however, differs entirely from electromagnetic waves and sound waves. In every nerve cell, there is a membrane separating the cytoplasmic fluid from the extracellular solution. The transmembrane voltage (also called membrane potential) is defined as the inside potential minus the extracellular potential. When the nerve fiber is at rest,the membrane potential is about -70 mV. The nerve impulse is a sharp change of the membranepotential. Therefore, it is also known as the action potential (Fig. 2.1).
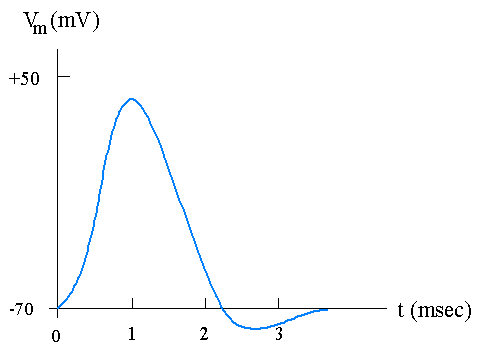
Figure 2.1. A typical nerve impulse (action potential).
The generation of action potentials is mainly due to the changes of sodium(Na+) and potassium (K+) conductances. Figure 2.2 shows the concentrations of Na+ and K+ ions on both sides of a nerve membrane. ForNa+, its concentration on the extracellular side is much higher than inside. We immediately notice thatNa+ ions are far from electrochemical equilibrium -- both the electric force due to electric potential difference and the chemical force due to ion concentration difference are pointing inward. How could the nerve membrane maintain such a stable state? This is because the conductance ofNa+ ions in the membrane is very small at the resting membrane potential. Although the inward driving force is large, the resultingNa+ influx is small. This small influx can be balanced by a slow ion transport process, theNa+-K+ pump, which moves Na+ ions outward and simultaneously K+ ions inward.
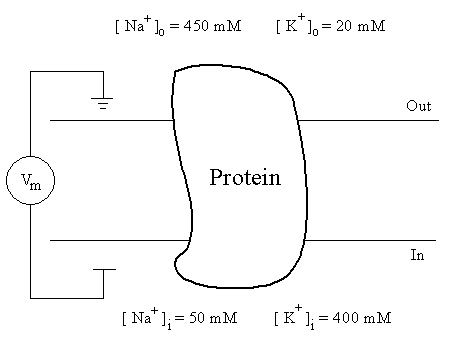
Figure 2.2. Ion concentrations on both sides of a nerve membrane, which contains various types of proteins. Ions may move across the membrane through a special class of proteins called ion channels.
The conductance of Na+ ions may change dramatically with the membrane potential as demonstrated by voltage clamp experiments, in which the membrane potential is displaced to a new value and maintained there (Fig. 2.3). Because ions carry charges, the movement of ions across the membrane will change the membrane potential. To maintain a constant membrane potential, the voltage clamp circuit must generate electric currents to neutralize the membrane potential change caused by the ionic flux. Thus, the ion current through the membrane is reflected in the electric current of the voltage clamp circuit outside the membrane.
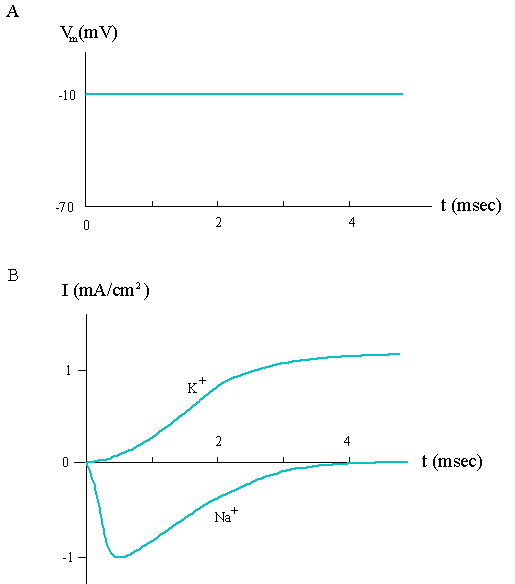
Figure 2.3. Ion currents measured from the voltage clamp circuit. (A) The membrane potential is depolarized from -70 mV to -10 mV at t = 0, and kept constant by the voltage clamp circuit. (B) The resultingNa+ and K+ currents through the membrane (outward positive) as measured from the voltage clamp circuit.
A membrane is said to be depolarized if the new potentialVm is more positive than the resting potential Vm0, and hyperpolarized ifVm < Vm0. Since depolarization makes the inside potential more positive, we expect (from the consideration of electrochemical forces) the currents of all cations to increase in the outward direction. Fig. 2.3 shows the experimental result for squid axons. TheK+ current indeed increases in the outward direction. However, the behavior ofNa+ current is totally unexpected. It first rises sharply in the inward direction and then declines with a slower rate to its resting value. Phenomenologically, the change of theNa+ current can be attributed to the change in Na+ conductance. Experimental results indicate that theNa+ conductance increases in the early stage of depolarization, which exceeds the reduction in the inward driving force. As time proceeds, theNa+ conductance decreases, resulting in the late decline of Na+ currents. The rise and fall ofNa+ conductance are known as sodium activation and sodium inactivation, respectively. They play important roles in the generation of a nerve "impulse", which must contain a rising phase as well as a falling phase.Mathematically, the ion conductance is defined by
Gion = Iion/(Vm -Vion) (1)
where Iion denotes the ion current through the membrane (outward current is defined as positive), andVion is the equilibrium potential for the ion. According to the Nernst equation,
Vion = (RT/F) ln([ion]o/[ion]i) (2)
where R denotes the gas constant, T is the absolute temperature, F represents the Faraday constant,[ion]o and [ion]i are the ion concentrations in the extracellular and intracellular fluids, respectively.
At room temperature, the equilibrium potential forNa+ with concentrations given in Fig. 2.2 is +55 mV. When the membrane potential is depolarized,Iion varies with time as shown in Fig. 2.3. From eq.(1), we can obtain the time course of the ion conductance. Fig. 2.4 shows the experimental results for a few depolarizations. We see that the peak ofNa+ conductance increases with increasing depolarizations before it levels off at large depolarizations. Another feature to remember is that the rising rate ofGK is much slower than GNa.
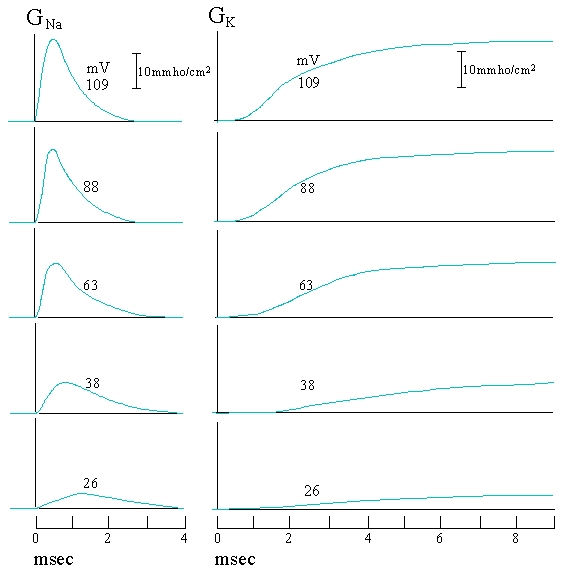
Figure 2.4. Time courses of Na+ and K+ conductances at a few depolarizations. The number next to each curve represents the depolarizing voltage from the resting potential -70 mV.
Font size:
Interval:
Bookmark:
Similar books «Molecular Mechanisms of Learning and Memory»
Look at similar books to Molecular Mechanisms of Learning and Memory. We have selected literature similar in name and meaning in the hope of providing readers with more options to find new, interesting, not yet read works.
Discussion, reviews of the book Molecular Mechanisms of Learning and Memory and just readers' own opinions. Leave your comments, write what you think about the work, its meaning or the main characters. Specify what exactly you liked and what you didn't like, and why you think so.